The Energy Crisis in Brain Cells: The Pivotal Role of Mitochondrial Dysfunction in Neurodegeneration
Modulating mitochondrial function is an important approach to treating neurodegenerative disorders. Indeed, researchers in both Industry and Academia are actively exploring pharmacological interventions focused on enhancing mitochondrial function through various pathways, such as elevating metabolic-related status, improving electron transport chain efficiency, recovering mitochondrial depolarization, decreasing oxidative stress, enhancing mitochondrial biogenesis, and promoting mitophagy. The MitochondriaWorld™ platform is an excellent resource that shows that both large pharma (e.g, Abbvie, Lilly, JnJ) and small biotechs (e.g. Mission Therapeutics, Pretzel Therapeutics, MitoRx Therapeutics) are actively pursuing therapeutic approaches to improving mitochondrial health.
Read on to find out about:
- The Central Role of Mitochondria in Brain Function
- Critical Role of Mitochondrial Health in Neuronal Survival
- Link Between Mitochondrial Dysfunction and Neurodegenerative Diseases
- Mitochondria as a Target for Therapeutic Intervention
- Advanced Assays for Mitochondrial Research
- Future Perspectives in Neurodegeneration Treatment
Mitochondria, an organelle crucial for brain function
The brain is indispensable to every aspect of human life, influencing our thoughts, actions, emotions, and physical well-being. It is the control centre for various bodily functions, including, but not limited to, motor function, sensory processing, broad body homeostasis (eg., body temperature, respiratory, and cardiovascular function), and endocrine system control. To carry out these functions, the brain requires continuous maintenance, adaptation, refinement, and, therefore, an energy source.
Mitochondria are the primary organelles responsible for energy production. Mitochondria are highly active organelles that can change their function and dynamically adapt to a cell’s metabolic needs. These properties of mitochondria are essential given their critical role in distinct mechanisms, including brain development, synapse formation, cellular differentiation, receptor anchorage, neurotransmitter vesicular transport, exocytosis, synaptic pruning, and neuronal plasticity.
Gaining insight into mitochondrial behaviour — both when they’re functioning properly and when they’re not (dysfunction) — is key for developing new treatments for age-related cognitive decline and neurodegenerative disorders. Given the high energy demands of neurons, they are particularly sensitive to changes in energy supply. A lack of sufficient energy can damage or lose function in these critical brain cells. Therefore, it’s crucial to understand how mitochondria contribute to this process.
Mitochondria: The powerhouses of the cell
Cells generate their primary energy source, adenosine triphosphate (ATP), in the mitochondria. These organelles play a crucial role in energy metabolism and are essential for the survival and functioning of nearly all eukaryotic cells.
Mitochondria produce ATP through a process called oxidative phosphorylation. In this process, several mitochondrial complexes, called the mitochondrial respiratory chain, are involved in the movement of electrons, leading to ATP generation and maintenance of the mitochondrial membrane potential (MMP). Maintaining the MMP is crucial in controlling intracellular calcium levels in neurons by absorbing excess ions and ensuring appropriate calcium balance.
In addition to generating ATP, mitochondria produce reactive oxygen species (ROS). While normal levels of ROS have signalling functions (such as in cellular proliferation, neuronal pruning, oxygen sensing and response to stress and inflammation), excessive production can lead to oxidative stress resulting in changes in MMP, calcium levels and energy production.
Mitochondria and Neurodegeneration
Neurons, highly specialized cells, must maintain their energy supply, calcium levels, and reactive oxygen species (ROS) balance to preserve their homeostasis and ensure survival. Disruption in calcium homeostasis, commonly associated with mitochondrial dysfunction, can lead to neuronal excitotoxicity and cell death. Additionally, oxidative stress, characterized by an imbalance between excessive ROS production and the cell’s antioxidant defences, is a prevalent factor in neurodegenerative diseases, contributing significantly to neuronal damage.
Given that in many neurodegenerative disorders, there is neuronal death, the role of mitochondria is pivotal (Figure 1). This includes disorders such as Alzheimer`s Disease (AD), Huntington`s Disease (HD), Parkinson`s Disease (PD) and Amyotrophic Lateral Sclerosis (ALS).
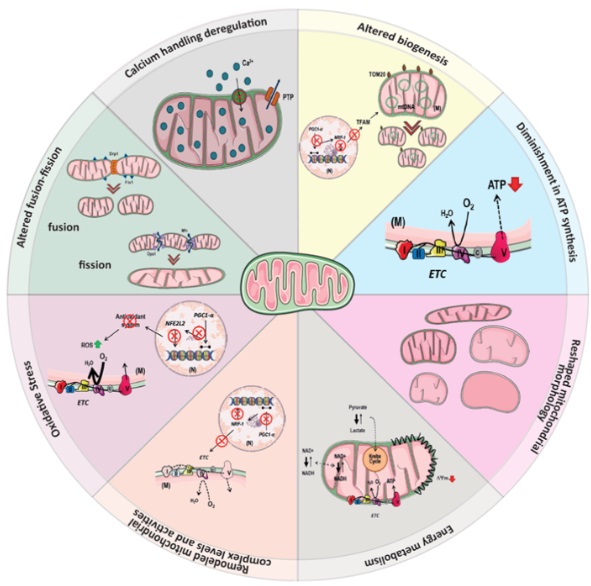
Figure 1: Schematic representation of the pivotal role of mitochondrial dysfunction in neurodegeneration. Mitochondrial dysfunction described in neurodegenerative diseases may be related to several factors, including increased oxidative stress, reduced mitochondrial complex levels and activity, dysregulation of Ca2+ homeostasis, reduced ATP levels, and changes in mitochondrial dynamics (fission and fusion processes), morphology, mtDNA copy number, and mass. Figure created in BioRender.
Mitochondrial Dynamics and Biogenesis
Mitochondrial dynamics, which include the processes of fusion and fission, along with biogenesis, play a vital role in preserving the health of mitochondria in cells. This is particularly important in neurons, which require large amounts of energy. The ability of mitochondria to merge (fusion) and divide (fission) ensures that they can maintain an optimal condition and quantity to meet the cell’s energy demands.
- Mitochondrial fusion allows for the exchange of genetic and protein material between mitochondria, promoting the mixing of healthy components and rescuing damaged ones.
- Mitochondrial fission, however, facilitates the removal of damaged or dysfunctional parts of mitochondria through processes like mitophagy (see below).
- Mitochondrial biogenesis, is the process by which new mitochondria are generated within cells. This involves the replication of mitochondrial DNA and the synthesis of proteins required for mitochondrial function. It is an essential mechanism for replenishing the mitochondrial pool.
It is well known that abnormalities in mitochondrial dynamics can lead to the accumulation of damaged mitochondria, increased oxidative stress, and neuronal damage. Reduced biogenesis may compromise the cell’s ability to replace damaged mitochondria, accumulating dysfunctional organelles. This decline in mitochondrial turnover contributes to energy deficits, impaired cellular function, and increased vulnerability to stressors, all of which are implicated in the pathogenesis of neurodegenerative disorders.
Understanding and targeting these aspects of mitochondrial function are active research areas for potential therapeutic interventions in neurodegenerative disorders. Strategies aimed at promoting mitochondrial health, mitophagy (see below), and biogenesis may offer promising avenues to slow the progression of these conditions.
Mitophagy: Quality Control of Mitochondria through Autophagy
Enhancing mitophagy has emerged as a potential therapeutic strategy for neurodegenerative diseases. This includes modulating vital regulatory proteins involved in this process. Mitophagy is a specific autophagy that explicitly targets damaged or dysfunctional mitochondria. The relevance of mitophagy to neurodegeneration is significant, as it plays a crucial role in maintaining mitochondrial quality and cellular homeostasis. Dysregulation of mitophagy may lead to the accumulation of defective mitochondria, contributing to the progression of neurodegenerative disorders. Of relevance, the accumulation of damaged mitochondria contributes to oxidative stress, impaired energy production, and increased vulnerability to cellular damage in neurons.
Several key proteins and regulatory pathways, including PINK1 (PTEN-induced kinase 1) and Parkin, orchestrate mitophagy. In Parkinson’s disease, for instance, mutations in such genes are directly linked to the disease, the accumulation of damaged mitochondria and the aggregation of alpha-synuclein. In Alzheimer’s disease, disruptions in mitophagy contribute to the accumulation of damaged mitochondria, leading to increased oxidative stress and neuronal dysfunction, and may contribute to the accumulation of beta-amyloid plaques.
Assays to Interrogate Mitochondrial Function
Given the fundamental role of mitochondria in cell health and the focus of mitochondrial-targeted therapeutics for treating neurodegeneration, high quality and robust assays that interrogate mitochondrial function are important. Examples of assays and endpoints that are necessary to understand mitochondrial function and health are listed below.
- ATP/ADP levels measurement
- NAD/NADH levels measurement
- Pyruvate/Lactate measurement
- Oxygen Consumption Rate
- Extracellular Acidification Rate
- Cytosolic calcium levels measurement
- Mitochondrial membrane potential measurement
- General oxidative stress levels measurement
- Mitochondrial superoxide levels measurement
- Lipid Peroxidation levels measurement
- Mitochondrial fission/fusion levels evaluation
- Mitochondrial Biogenesis levels evaluation
- Mitophagy
Levels of the main metabolites related to mitochondrial energy
By monitoring specific metabolites, we can infer the mitochondrial respiratory chain’s efficiency and the cells’ overall metabolic state, since the primary function of mitochondria is to generate the energy necessary for various cellular activities. This information can be crucial for understanding disease mechanisms, especially in neurodegenerative diseases where mitochondrial dysfunction is a hallmark, and for evaluating the potential efficacy of new therapeutic compounds targeting cellular metabolism.
To assess the bioenergetic health of cell cultures, the levels of several key energy-related metabolites can be measured (Figure 2). These include:
- ATP: The direct source of energy for cellular processes. Its abundance gives us a snapshot of the cell’s immediate energy supply.
- NADH (Nicotinamide adenine dinucleotide): A coenzyme that plays a critical role in energy production. It is a major electron donor in the production of ATP and serves as an indicator of oxidative stress and the metabolic state of the cell.
- Pyruvate: A key molecule in several metabolic pathways, but most notably, it marks the end product of glycolysis. In the presence of oxygen, pyruvate enters the mitochondria to be further processed for ATP production.
- Lactate: Typically produced from pyruvate under anaerobic conditions (when oxygen is limited). Lactate levels can indicate a shift in cellular metabolism, such as when cells rely on glycolysis instead of oxidative phosphorylation for energy production.
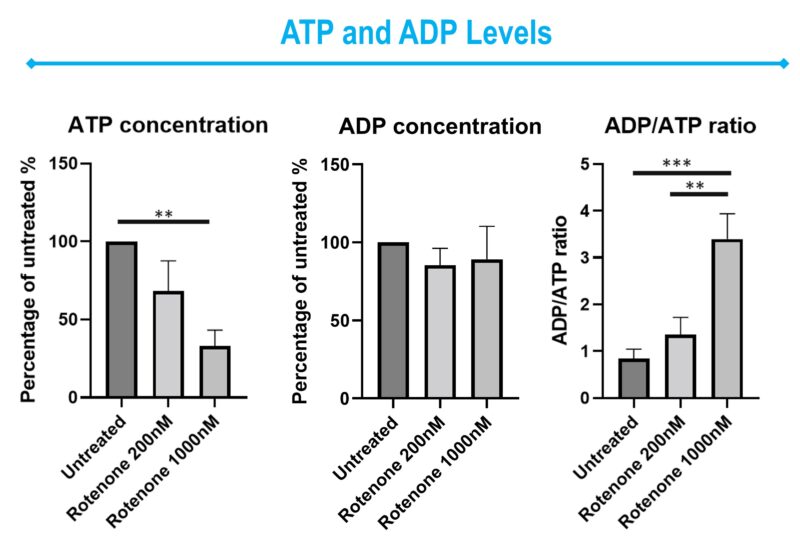
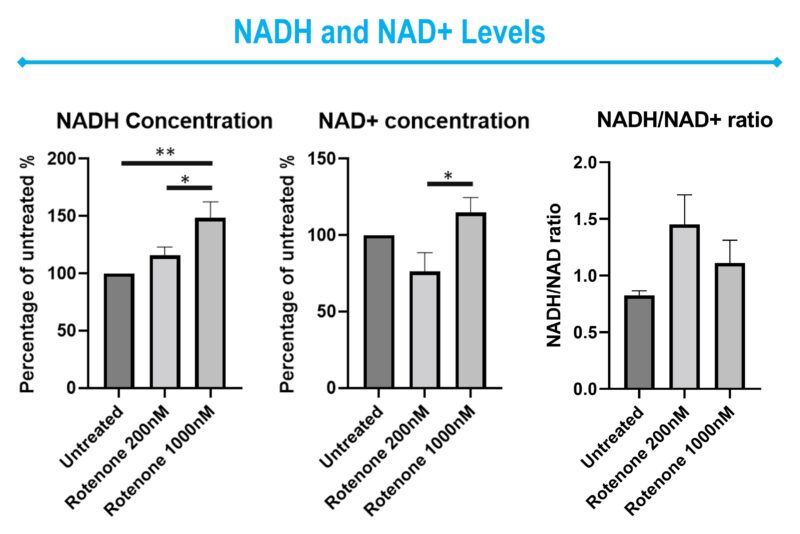
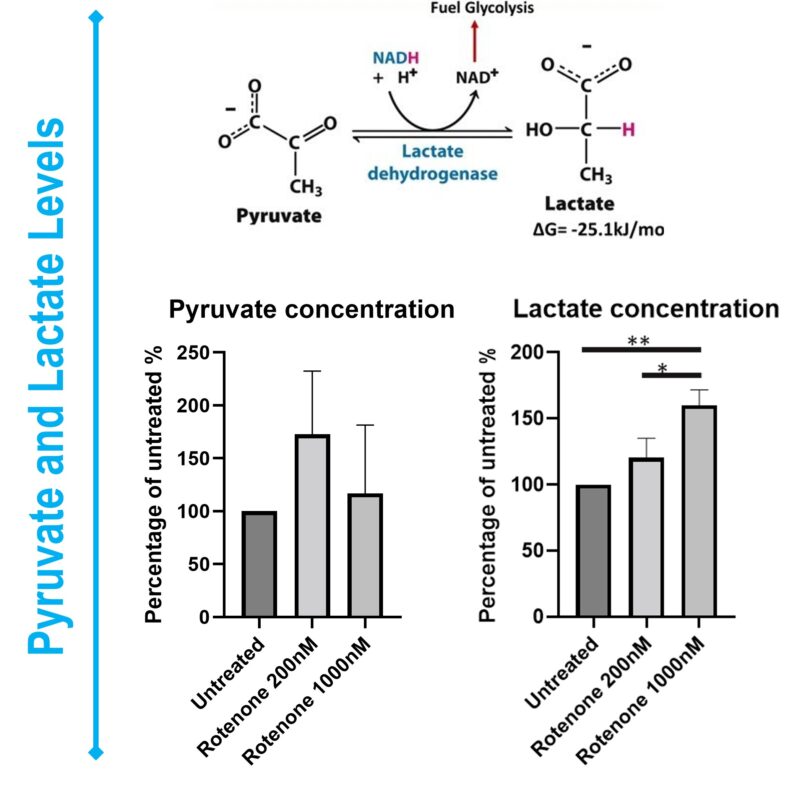
Figure 2: Representative bar graphics of ATP, ADP, NADH, NAD+, Pyruvate and Lactate levels on Rotenone-treated SHSY5Y cells. Data was normalized Data was expressed as a percentage of the control group.
Oxygen Consumption Rate and Metabolic status
Mitochondria produce ATP through a series of chemical reactions known as cellular respiration, which includes the electron transport chain. The efficiency of these processes is critical for maintaining cellular homeostasis that allows cells to live and function. Importantly, changes in mitochondrial membrane potential, calcium homeostasis, and oxidative stress are frequently associated with alterations in cellular respiration.
The Seahorse instrument measures Oxygen Consumption Rate (OCR). This platform allows the measurement of several mitochondrial respiration steps, namely basal respiration, maximum respiration and spare respiratory capacity (Figure 3).
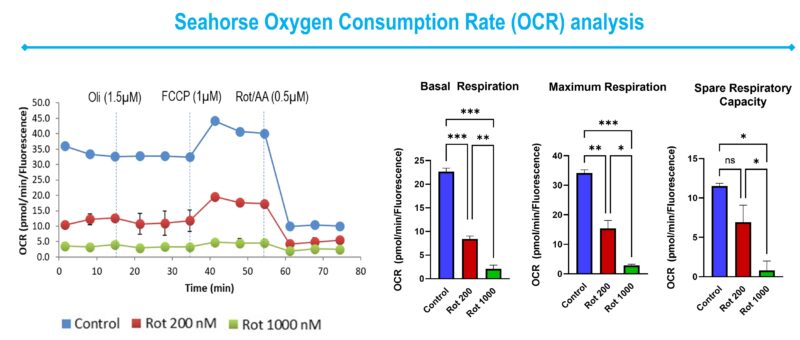
Figure 3: Representative line and bar graphics of Oxygen Consumption Rate (OCR) on Rotenone-treated SHSY5Y cells. As recommended, different pharmacological agents were injected throughout the assay, named Oligomycin (Oli) (1.5 µM), FCCP (1 µM), and a mix of Rotenone and Antimycin A (Rot/AA) (0.5 µM). OCR was normalized by cell number after cellular incubation with DAPI.
Assessing Mitochondrial Functional Parameters via Kinetic Experiments
In mitochondrial research, kinetic experiments play a pivotal role in unveiling the complexities of mitochondrial function. These assays, characterized by their robustness, reproducibility, and validation, are instrumental in assessing the dynamical functional parameters of mitochondria. Employing fluorescence probes adaptable across diverse plate reader platforms, we can meticulously track changes in mitochondrial behaviour over time (Figure 4). This method enables the precise evaluation of mitochondrial functional status of healthy (control) and disease-affected cells (Figure 4).
It is crucial to optimize each probe for use in different cell types, and to include reference compounds, such as carbonyl cyanide 4-phenylhydrazone (FCCP) (Figure 4). FCCP is a mitochondrial protonophore, which can depolarize the mitochondrial membrane potential, releasing mitochondrial calcium into the cytosol, and inducing an increase in oxidative stress.
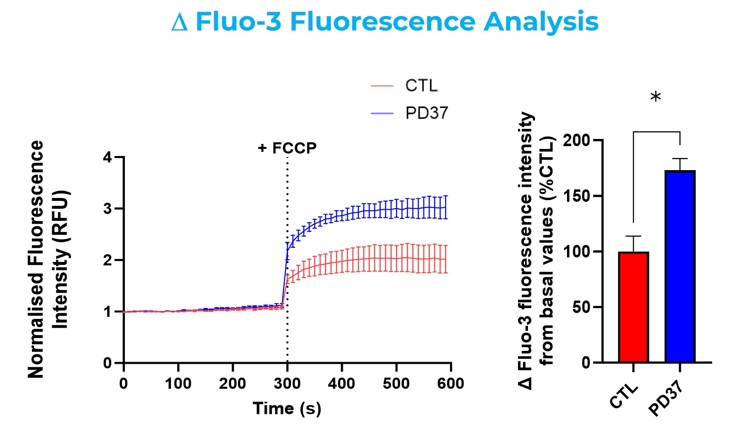
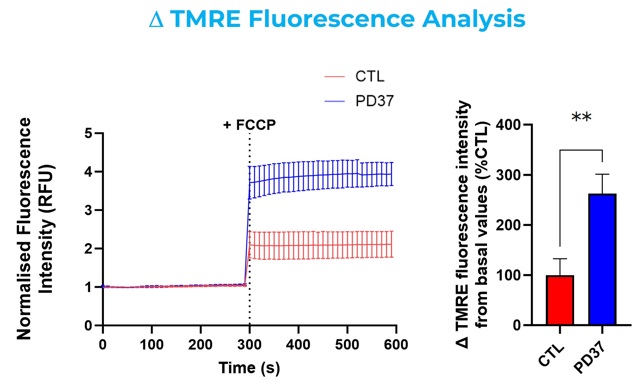
Figure 4: Representative line and bar graphics of control (healthy) and diseased fibroblasts incubated with Fluo-3-AM and TMRE. Kinetics readings in the absence and presence of FCCP. Data was expressed as a percentage of the control group. PD37: Parkinson`s Disease cell line.
By quantifying these temporal changes, our approach provides a comprehensive understanding of mitochondrial functionality under various physiological conditions, offering critical insights for advancing our knowledge of mitochondrial contributions to cellular health and disease.
Conclusion: Navigating the Future of Neurodegeneration Treatment through Mitochondrial Health
As we delve into the complexities of neurodegenerative diseases, it becomes increasingly clear that mitochondria play a central role in the vitality of brain cells and the pathogenesis of these conditions. Our exploration of mitochondrial function, dynamics, biogenesis, and quality control mechanisms, underscores the intricate link between mitochondrial health and preserving neuronal integrity.
As highlighted in resources like the MitochondriaWorld™ platform, the burgeoning field of mitochondrial research reveals a concerted effort by the scientific community to uncover targeted therapies that can restore or enhance mitochondrial function. This endeavour is not just about combatting the symptoms of neurodegeneration but fundamentally understanding and addressing the underlying mechanisms that lead to neuronal decline.
Moreover, developing and utilising sophisticated assays and techniques for mitochondrial analysis signifies a pivotal shift in our approach to neurodegenerative disease research. By assessing mitochondrial parameters, such as ATP production, oxygen consumption and oxidative stress levels, we deepen our understanding of these diseases and pave the way for novel therapeutic strategies.
We remain hopeful as we continue to unravel the mysteries of mitochondrial function. The collaborative efforts of researchers and the ongoing advancements in mitochondrial science hold promise for developing innovative, effective treatments that can significantly improve the lives of those affected by these debilitating conditions.
About the author
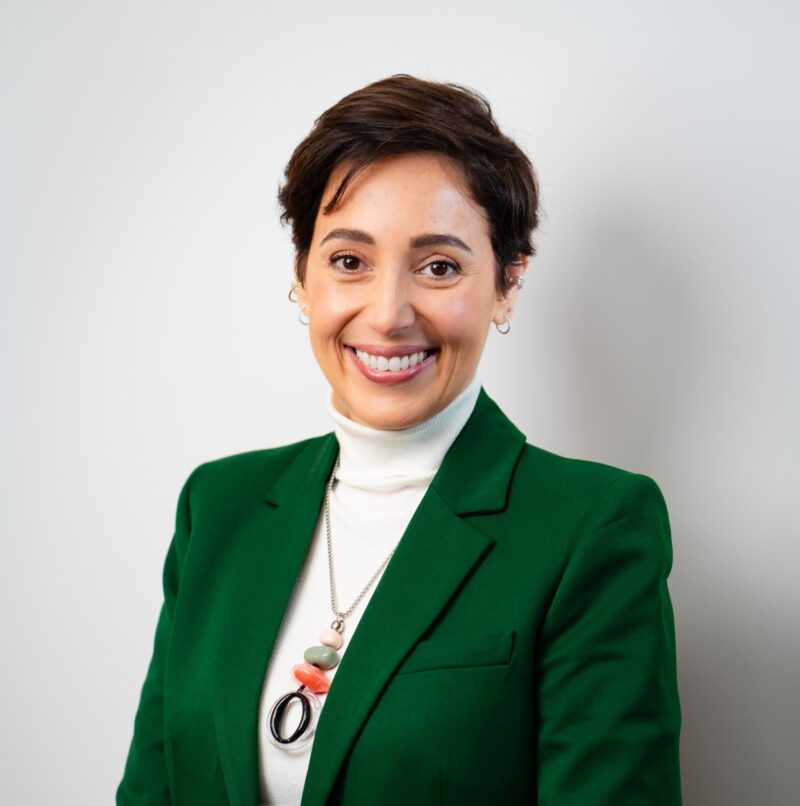
Follow Sygnature Discovery on LinkedIn to watch Tatiana’s 5 part video series, #MitochondriaUnlocked
Tatiana Rosenstock has had an exceptional career and has made significant contributions to the field of Neuroscience for 20+ years. Her work has focused on topics such as mitochondrial deregulation, transcription deregulation, and autophagy in neurodegenerative diseases, such as Huntington’s Disease, and Amyotrophic Lateral Sclerosis, and Schizophrenia.
At Sygnature Discovery, Tatiana is a Principal Scientist overseeing various neuroscience-related projects in different sub-areas such as metabolism (mitochondrial function), biomarkers, microglial function and activation, and neuroplasticity. During this period, she has been working with distinct in-vitro models, named iPSC-derived neurons, primary cortical neurons, Alzheimer`s and Parkinson`s disease fibroblasts, neuroblastomas cell lines, tissue and biofluids from transgenics animal models of Alzheimer`s disease.